- Solar energy blog
- Lifecycle analysis of a PV plant: Carbon footprints and recycling modules
Lifecycle analysis of a PV plant: Carbon footprints and recycling modules
Maïlys Babin
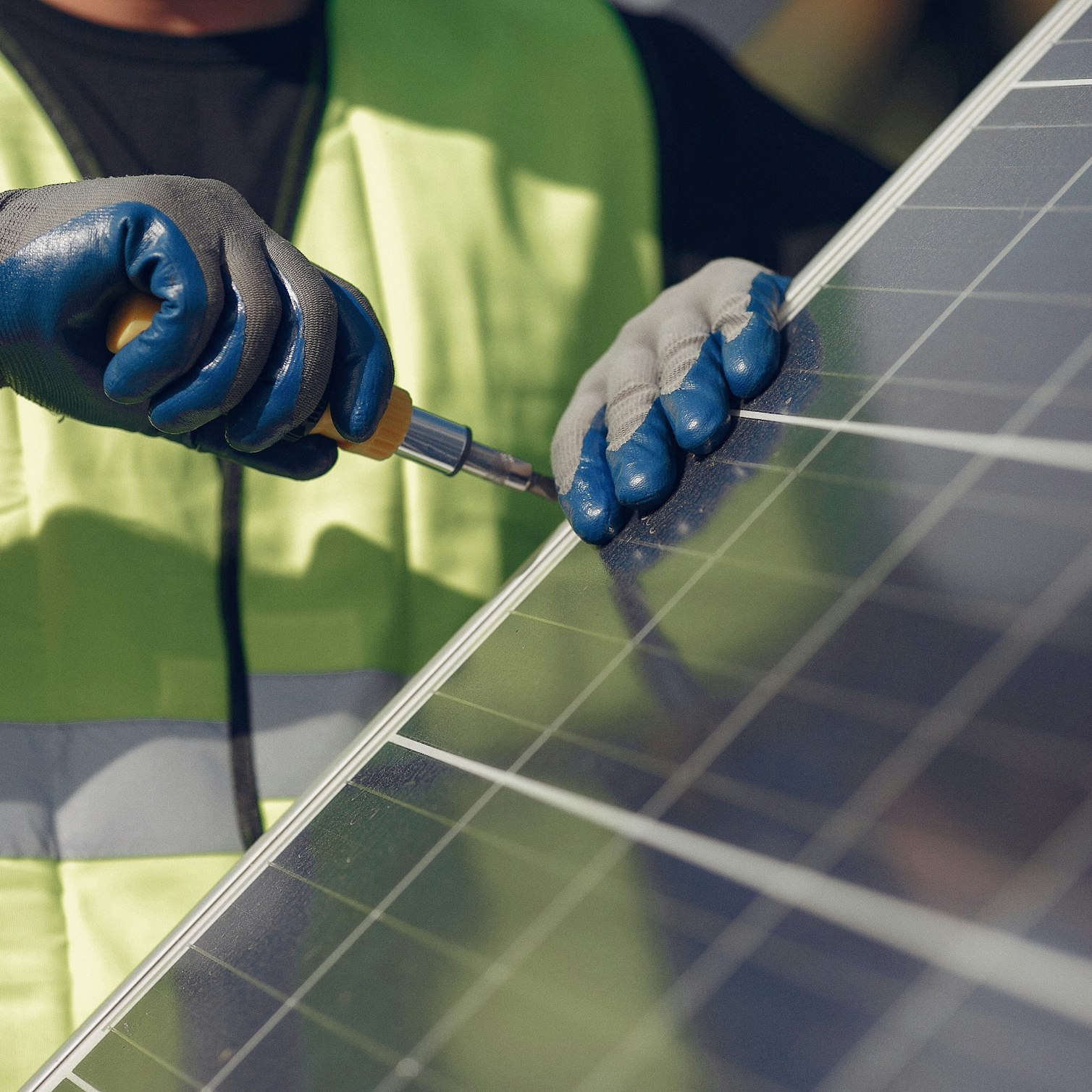
Content
Lifecycle analysis (LCA) of electricity generation projects is an essential stage of the planning process to evaluate their environmental impact. LCA examines the inputs and outputs of a project during its full lifecycle — from planning to decommissioning — and is used to formulate energy policy and make decisions about research and development (R&D) funding.
LCA is a valuable tool for PV system optimization based on a range of environmental variables. It also provides a means to directly compare different types of energy technologies, demonstrating how investing in a PV system compares with other forms of electricity generation.
So, how does lifecycle analysis work for solar photovoltaic (PV) plants?
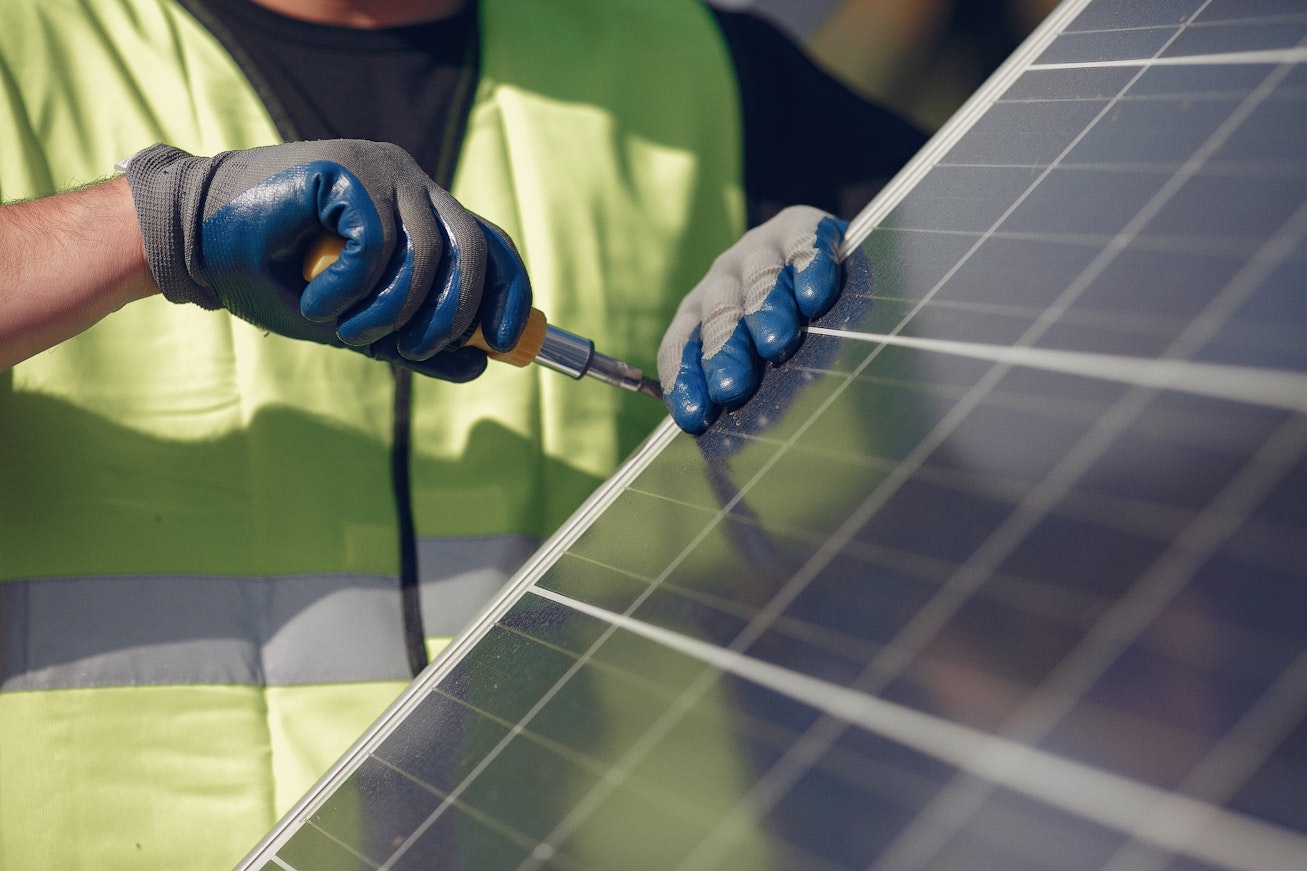
Lifecycle analysis of a PV plant
The useful life of a PV system is estimated to be 25-40 years, depending on factors such as the equipment used and environmental conditions. LCA of a PV system looks at the impact on the environment from the production of equipment through to the disposal of the panels. The lifecycle stages of photovoltaics involve:
raw material extraction
raw material processing and refining
manufacturing of PV modules and other system components
installation
system operation and maintenance
plant decommissioning and disposal or recycling
Let's take a look at the key phases in more detail.
Raw materials and resources
LCA starts with inventory analysis to evaluate the volume of materials a project will use that have an environmental impact and whether there will be any environmentally damaging outputs, such as carbon dioxide (CO2) emissions, during a plant’s lifecycle.
Raw materials such as metals are associated with emissions generated from the extraction and then transportation processes involved in their production.
The processes throughout the lifecycle are evaluated quantitatively and the data is analyzed to identify all of the materials involved. It is important to understand the quality of the data and the assumptions involved, as it can be challenging to collect comprehensive information on all related processes — resulting in missing or simplified data.
In addition to the environmental impact of the extraction of raw materials, it is also important to take into account the scarcity of these resources. For instance, PV installation requires aluminum, copper, steel, concrete, silver, silicon, and graphite.
Copper for instance is now a very strategic resource with extra vigilance required regarding its supply. IFPen estimates that cumulative global copper consumption by 2050 could exceed the level of known resources in 2019.
Currently, the leading technology for PV modules is silicon. At a global level, the accelerated development of photovoltaic installations is leading to significant quantities of silicon being used. Unlike other resources, silicon is not geologically scarce. Nevertheless, the supply of silicon for photovoltaic applications is a matter of particular concern, and a political issue linked to the strong dependence of silicon supply to China, which dominates the silica production market
Recycling
Recycling of the raw materials used in PV panels and systems is becoming increasingly important as the clean energy transition progresses. The disposal of PV panels in landfills will become a bigger environmental issue as more systems reach the end of their lifespan, all while new sources of raw materials will need to be excavated in order to meet demand.
The global supply of copper — which is used in solar panels and electrical wiring, as well as wind turbines, nuclear plants, energy storage batteries, and power grids — is expected to tighten, as it is also in high demand for infrastructure such as buildings, transport, and telecom networks.
While demand is expanding in developed and developing economies, there are few new mining projects in the pipeline aimed at ramping up copper refining to keep up with the growth in consumption. The larger the share of renewable generation in the energy mix, the more copper will be required.
The supply chain will need to respond by building out recycling capacity to increase the availability of copper material from used panels and other equipment, without new primary production capacity. Tighter supply will likely lead to increased raw material costs, making recycling more financially viable but also increasing system costs.

There are similar concerns about the availability of silicon used in crystalline solar cells. While there is silicon refining capacity spread around the world, the refining process is highly energy intensive — which can make it unprofitable to produce when electricity prices are high. New silica mining will also be needed to support solar power growth and prevent a monopoly on supply.
The supply of lithium used in storage and electric vehicle batteries is also expected to become increasingly tight as mining is concentrated in Australia, Chile, and Argentina, while refining and battery production is centered in China.
Lifecycle analysis of fossil fuel energy vs renewable energy
How does the carbon footprint of renewable generation compare with fossil fuel generation? Does the production of equipment such as solar panels and wind turbines have a smaller or greater environmental impact than a fossil fuel plant?
The greenhouse gas emissions over the full lifecycle of a power plant are both direct and indirect. In the initial stages, indirect emissions come from the extraction, manufacturing, and transportation of raw materials and fuel, as well as the construction of electricity infrastructure. There are direct emissions from combustion in fossil fuel generation plants, while renewable sources do not produce emissions from generation. And then there are indirect emissions from the dismantling of electricity infrastructure at the end of its lifespan and the disposal of fuel as waste.
According to a report by France's electricity grid operator RTE, even when the full lifecycle of raw material extraction and processing, equipment manufacturing, installation, and maintenance is taken into account, the total CO2 emissions produced by renewable and nuclear generation technologies are much lower than those produced by oil, coal, and gas plants.
Among renewable technologies, solar PV panels have a slightly larger carbon footprint than nuclear plants or wind turbines. This is illustrated in the graph below showing life cycle emissions for different forms of renewable energies. In fact, nuclear plants do not directly produce any greenhouse emissions but the transport and disposal of waste products are currently powered through the use of fossil fuels.
The carbon footprint of PV plants could be reduced further if panel production is localized in order to reduce transport emissions, if there are advances in the types of panels used, a reduction of the amount of energy used for the production of the modules, or if the recycling of panels becomes standard.

Source: RTE France, Futurs énergétiques 2050: les scénarios de mix de production à l’étude permettant d’atteindre la neutralité carbone à l’horizon 2050.
LCA allows a holistic overview of the environmental impact of PV
LCA is one of the best approaches to have a complete overview of the environmental impact of PV as it covers every step of the project, from raw materials to decommissioning. Nevertheless, it is important to keep in mind that it is a very complex evaluation with some inherent uncertainty. Additionally, the results presented here are dependent on the evolution of the process and thus will change over time.
For a complete overview of the environmental aspect, other aspects might be considered, such as occupation and use of land, air pollutants, health impact, etc.
LCA can help make the case for solar as an effective alternative to fossil fuel generation, helping to advance the transition to low-carbon energy.
For more insights on the energy transition, keep reading the RatedPower blog and visit our resources section.
2025 Trends: Renewable Energy & Solar Research Report
Get key insights and data from an industry-wide survey and solar simulations on the RatedPower platform. Download now to uncover critical trends and challenges shaping the future of renewables.
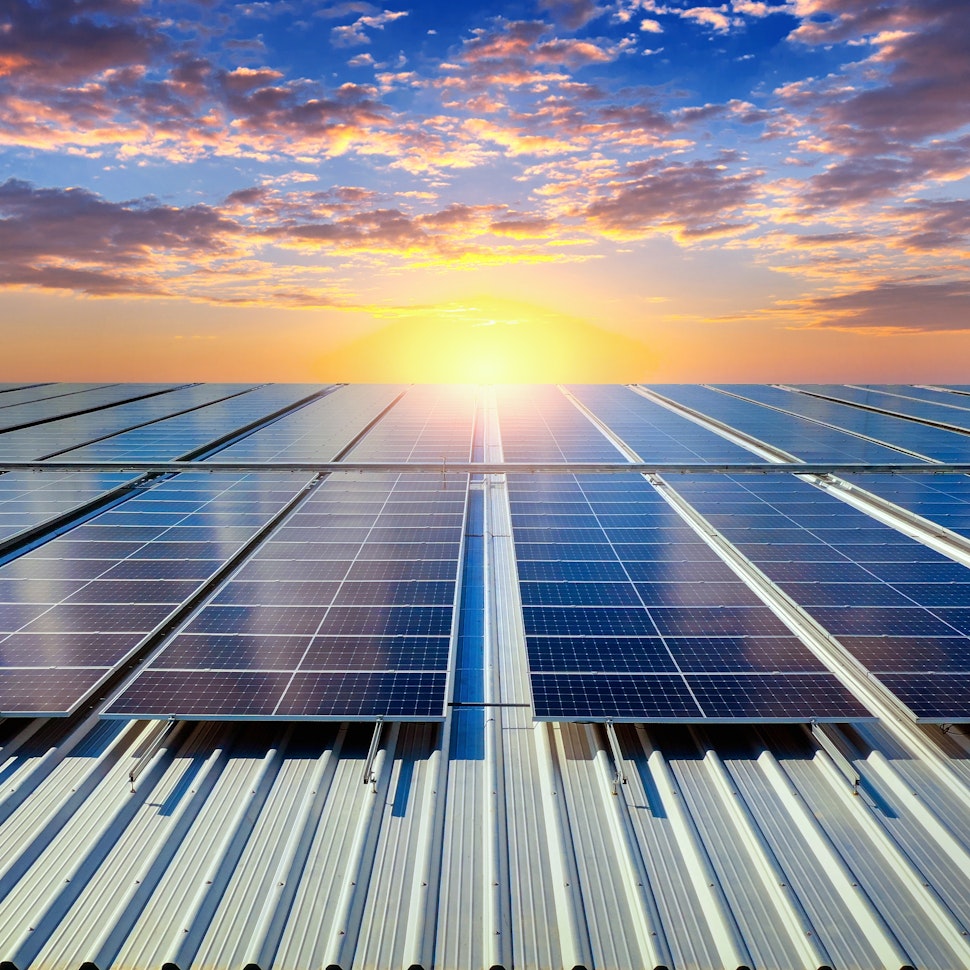
Latest stories
Related posts
Technology and engineering
Innovation in renewable energy: Developments expected in 2025
We look at the 10 biggest renewable industry developments that are making a green future possible, including perovskite solar cells, green hydrogen, and more.
Updated 18 MAR, 25
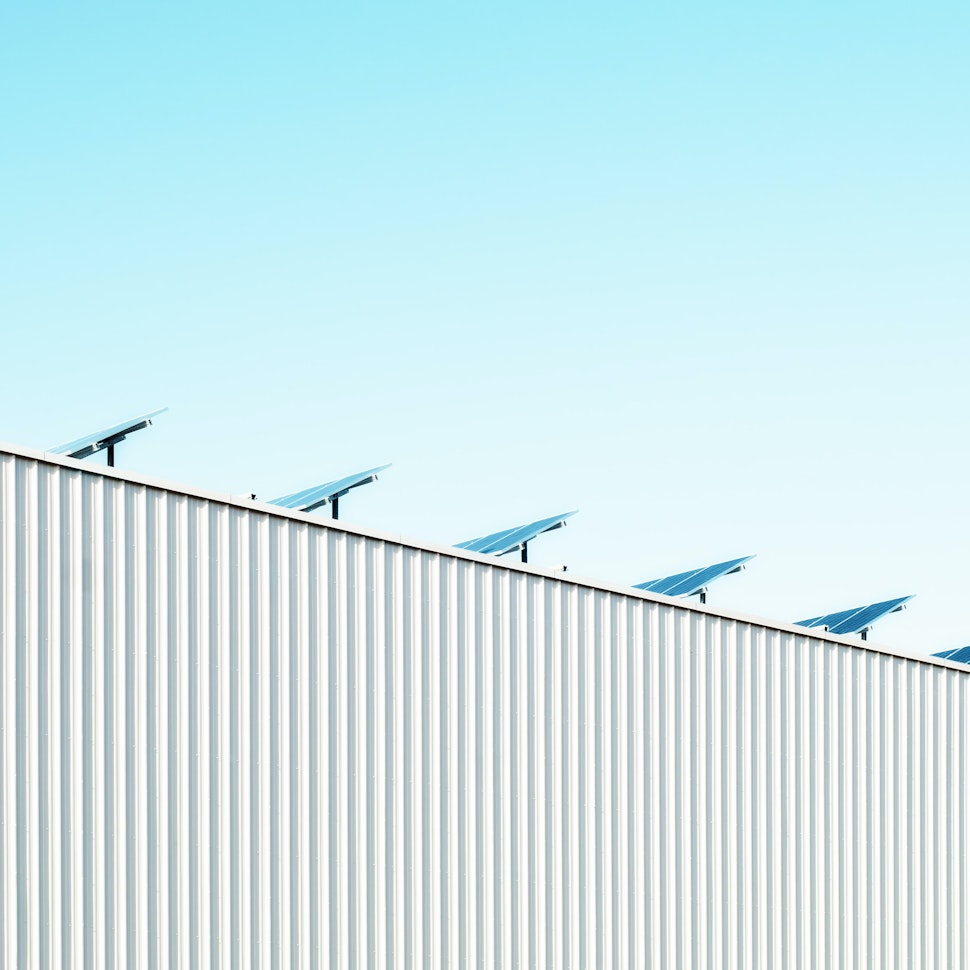
Market analysis
Solar energy in Australia: a 2021 market analysis
Updated 11 MAY, 21
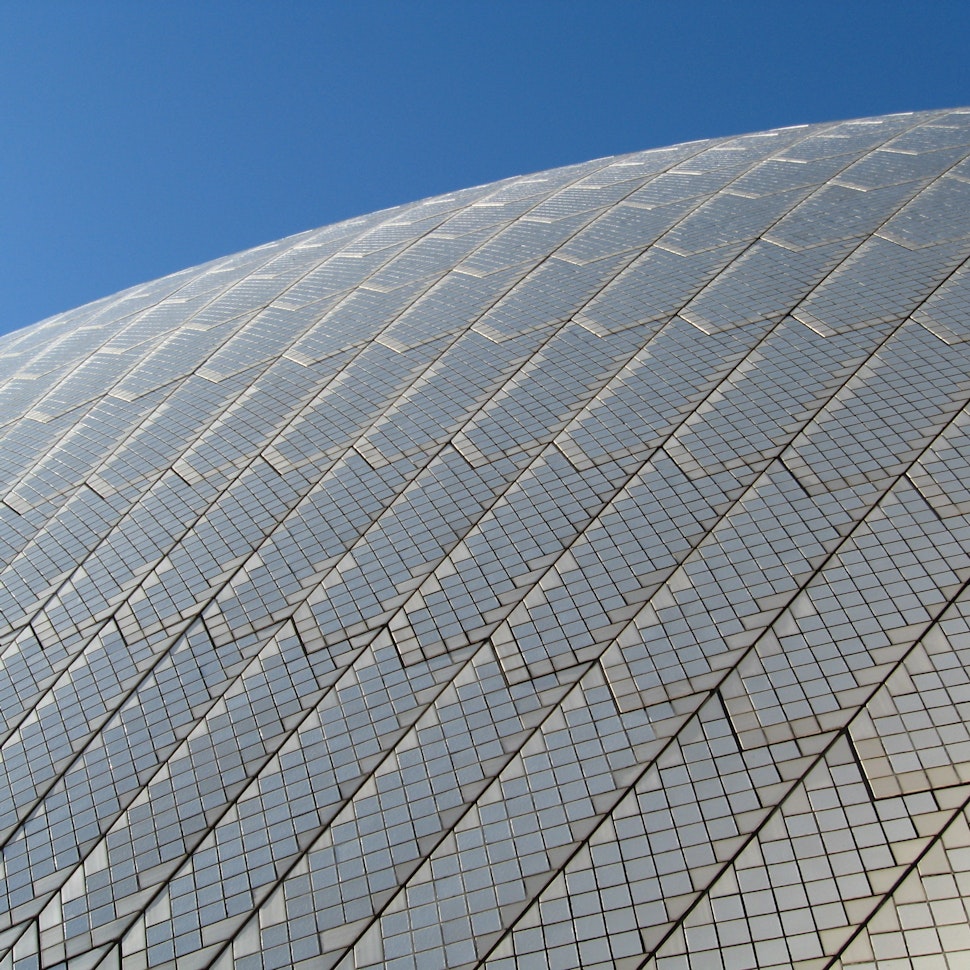
Technology and engineering
The era of standalone energy yield software is ending
Updated 19 APR, 22
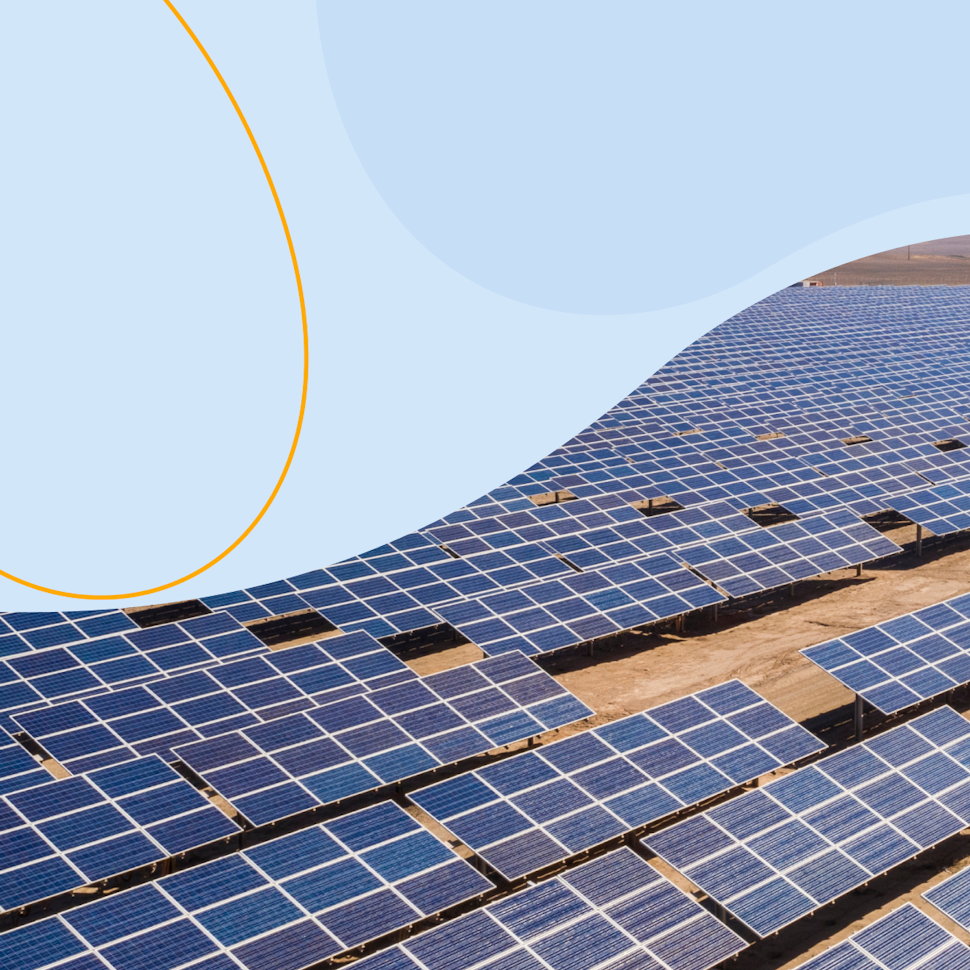
- RatedPower
- Solar energy blog
- Lifecycle analysis of a PV plant: Carbon footprints and recycling modules